The economics of fish farming and fish welfare in Europe
- Ren Ryba
- 3 days ago
- 24 min read
Updated: 16 minutes ago
A systematic review
Author: Ren Ryba, PhD
Download PDF Version
Executive summary
Several organisations, including Rethink Priorities, Welfare Footprint, Animal Ask, and others, are conducting a year-long project to guide the strategy of the fish welfare movement for the coming decades. Building on previous work's interest and work in European farmed fish welfare by advocacy groups and expert scientists.
As part of this project, Animal Ask conducted a systematic review on the economics of fish production in European aquaculture. Our database searches resulted in over 23,000 scientific publications and industry, government, and NGO reports from countries across Europe. After scanning the title and abstracts of all of these, we extracted economic data and other insights from around 600 publications.
This report contains the detailed results from this review. We provide economic data on many aspects of salmonid, bass and bream, and carp production across Europe. We focus on levels of profitability, drivers of production costs, price transmission, and market power.
Key findings are as follows:
Profitability: There is large variation in profitability across species, countries and farms, and even within farms over time. That said, fish grow-out seems to be mostly profitable, indicating that many farmers may be capable of incurring additional costs for fish welfare improvements. Fish hatcheries/nurseries also appear to have healthy profit margins. There are some specific industries that have low or negative profitability.
Costs of production: The largest cost of production is usually feed, followed by labour and stocking. This indicates that increases in feed prices are most relevant for market prices for some fish products (see next dot point). Again, there is large variation across contexts.
Price setting: For Atlantic salmon, farmers tend to have power to set prices, but retailers also have some power in large consumer markets (e.g. UK, France, Germany). For bass and bream, retailers generally set prices, and farmers are forced to accept these prices. This indicates that retailers may have more latitude for setting fish welfare policy for European seabass and gilt-head seabream and in some large salmon markets.
Market integration: Farmed salmon has many substitutes, including wild-caught salmon and other wild-caught species. Farmed bass and bream tend not to be substitutes with wild-caught bass and bream. However, this varies across different markets. This might indicate that consumers would respond to price increases by switching to other types of fish for salmon. This substitution is less likely for bass and bream consumers, though consumer responses are not completely known.
Response to price changes: Demand for salmon is relatively unresponsive to price in the short run but comparatively more responsive to price in the long run. This indicates that increasing the retail price of salmon may have limited impact on the number of salmon farmed, at least in the short term.
Costs of welfare improvements: There is very little evidence on the economic effects of fish welfare improvements. The only studies that have examined this question have been hypothetical simulation studies, not analyses of welfare improvements in the real world. This is a key knowledge gap.
The studies in our review also provide many qualitative insights that enrich our understanding. Some particularly interesting findings are:
Inflation and global instability (e.g. due to the COVID-19 pandemic and Russia's invasion of Ukraine) has appeared to cause a net decrease in fish consumption by volume in Europe. Likewise, political conflict between China and Norway led to import restrictions against Norwegian salmon sold in China.
The market share of certified fish (e.g. Aquaculture Stewardship Council) is generally small, but meaningful in some contexts (e.g. 6% of trout sold in Germany). Where farmers seek certification, this is largely driven by market access and industry reputation. Some farmers also observe higher market prices after obtaining certification.
Production in alternative systems (e.g. organic, integrated multi-trophic aquaculture) is very low.
We have also included special sections in this report on juveniles, cleaner fish, and sturgeon production, as well as some details of key biological statistics of production (e.g. harvest weights, duration of production, and mortality rates).
Table of contents
Acknowledgements
References
1. Economic findings
In this section, we summarise the key economic findings relating to aquaculture in Europe.
We advise that our economic results should be interpreted with caution for several reasons:
There is immense variance across different farms and even within farms over time. A single industry contains very profitable farms, very unprofitable farms, and farms in the middle; a single farm may be profitable in one year but unprofitable the following year. We're only showing average trends, and there will be individual farms that diverge significantly from these trends.
The literature did not contain economic data for every industry (combination of country and species group). However, most countries and most species were present in the data, even if not always in every combination.
The sources reported economic statistics in conflicting ways. For example, some sources reported gross profit rather than net profit; likewise, some sources excluded capital costs from the costs of production, and sources differed as to their inclusion of government subsidies as a source of farmer revenue. There is no way around this except to acknowledge that this variation adds additional noise to these already noisy statistics.
Note that sections 2.1.1 and 2.1.2 report statistics for grow-out only. Juvenile fish, as well as the unique industries of cleaner fish and sturgeon production, are instead addressed in section 2.2. This is due to limited data and the unique nature of these industries.
1.1. Profitability for grow-out
Profitability gives us information about how realistic it is for fish farmers to afford additional up-front investments or ongoing costs, which are sometimes required for fish welfare interventions.
Figure 1 shows the profitability of grow-out farms in aquaculture. The graph also shows the sample size or coverage of the aggregate data that we used to make each estimate; generally speaking, statistics that are derived from a larger sample of farms are more reliable.
For the purposes of Figure 1 only, we have not given the specific magnitude of profitability for unprofitable farms; we have scaled them all to the same amount. This is solely to assist with visualisation and to avoid a few very unprofitable farms from stretching the graph.
Profitability is expressed as the ratio of profit-to-revenue, which enables comparison across currencies and industries. A positive profit-to-revenue (to the right-hand side of the graph below) indicates that an industry is making more money than it spends, while a negative profit-to-revenue (to the left-hand side of the graph below) indicates that an industry is losing money. Even if an industry is profitable, a small profit-to-revenue indicates that profit margins are tight and that farmers might have difficulty making additional investments.
Most industries in our data are profitable and making money. Most of the economically significant industries are profitable (e.g. Turkish bass and bream; Norwegian salmon). In some cases, the profitability is quite high—this indicates that some industries may have the financial capacity to make additional investments in capital, as required for some types of fish welfare improvements.
In contrast, some industries are losing money. This is most notable for smaller-scale industries, especially carp and, apparently, anything to do with Germany.
It is not very meaningful to talk about averages for a species group as a whole. For example, calculating an average profitability for all salmonids requires combining data from different countries which have industries of different sizes and data of different quality and coverage. However, it might help to know some approximate averages simply as a mental guide. The median profitability, naively counting each country in the graph below as a single data point, is as follows:
Bass and bream: 7.2%
Salmonids: 13%
Carp: 0.6%
We emphasise once again that those three averages are highly fraught and only useful as a mental guide.
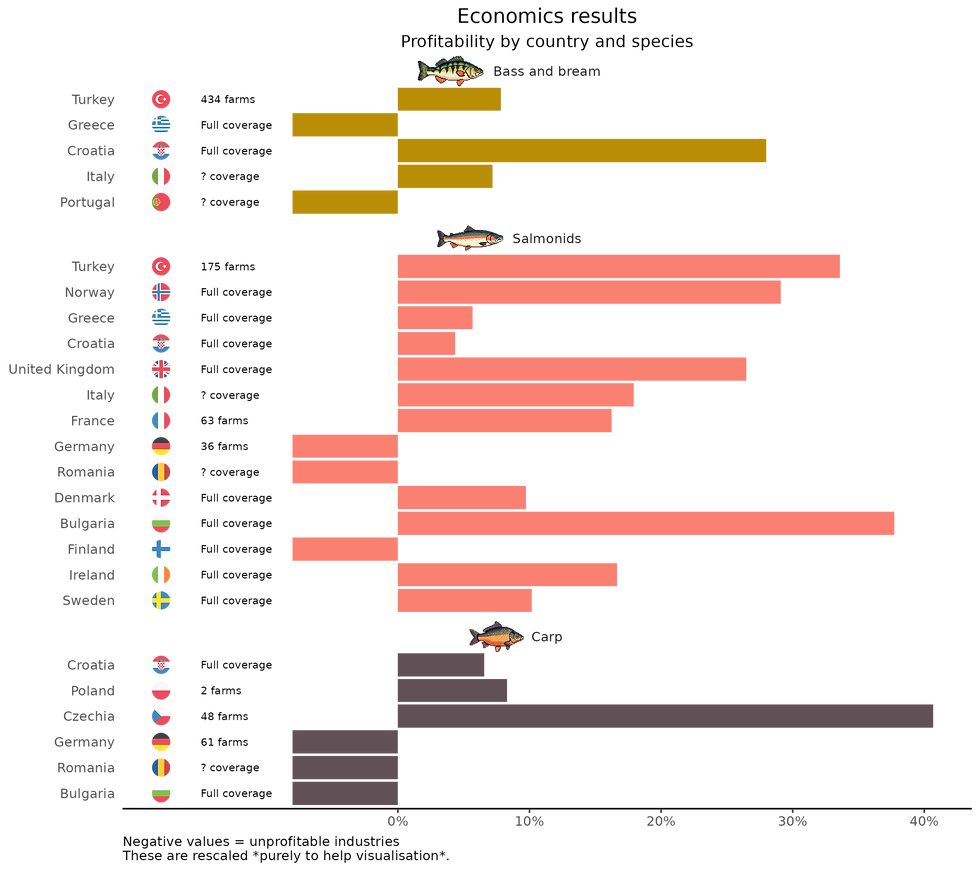
1.2. Cost breakdowns for grow-out
The cost breakdown gives us information about what is driving the costs facing fish farmers. This, in turn, can help us understand how the costs facing fish farmers might evolve over time or be affected by external factors.
Figure 2 shows the cost breakdown of grow-out farms in aquaculture. Again, the graph also shows the sample size or coverage of the aggregate data that we used to make each estimate; generally speaking, statistics that are derived from a larger sample of farms are more reliable.
In most cases, the largest cost component is feed. Labour and stocking are the other two large cost components. However, a large part of the cost of most industries corresponds to other cost components. This "other" category consists of a variety of small cost components, such as electricity, license fees, maintenance, water, depreciation, and so on.
Many of the carp industries have only a small proportion of their cost coming from feed and labour. This is usually the case for small-scale farms, perhaps run by an individual or a family, where carp may derive some of their nutrition from foraging rather than feed. In contrast, the more industrialised industries such as Norwegian salmon pay a large amount for commercial feed and paid labour.
In some industries, it is common to produce juvenile fish on-farm rather than purchasing juvenile fish from commercial hatcheries or nurseries. This explains why some industries have very low costs of stocking.
Like we mentioned above, it is not very meaningful to talk about averages for a species group as a whole. However, it might help to know some approximate averages simply as a mental guide. The mean cost components, naively counting each industry in the graph below as a single data point, are as follows:
Feed: 35%
Stocking: 11%
Labour: 14%
Other: 39%
We emphasise once again that those three averages are highly fraught and only useful as a mental guide.
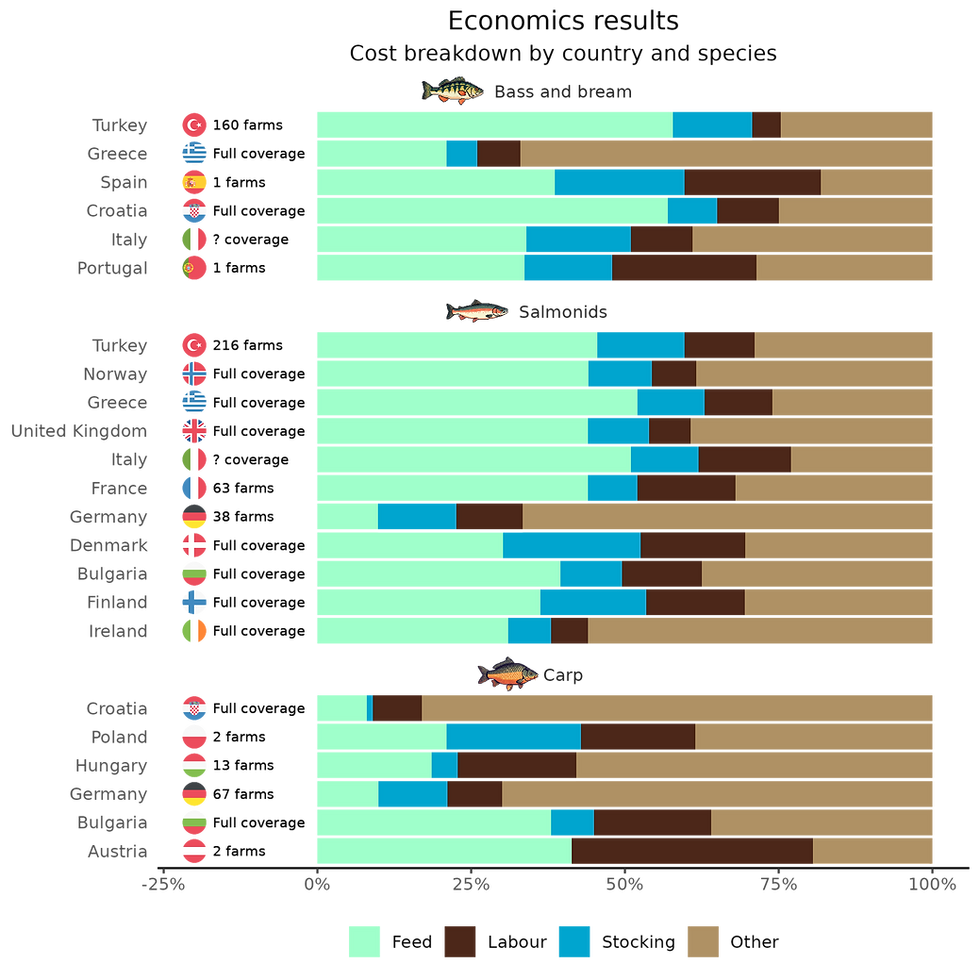
1.3. Price transmission, price-setting, and market power
Price transmission and market power matters because this gives us information about which members of the supply chain have power over retail prices. For example, if retailers have lots of market power and charge a heavy premium for a particular fish product, then they might be more willing to demand that producers meet comparatively expensive welfare improvements. On the other hand, if retailers charge a very thin premium for a particular fish product, then their power to set fish welfare policy might be more limited.
Figure 3 shows the price transmission across 15 case studies. Across these studies, the average share of retail price is as follows:
Ex-farm (price when leaving the farm): 47%
Added by distributors: 22%
Added during retail: 24%
Value-added tax (VAT): 8%
This data does not let us compare the price transmission across species groups, as the methodology was slightly different for different species groups.
Note that, in the graph, all salmonids are rainbow trout. We found no such case studies on Atlantic salmon.
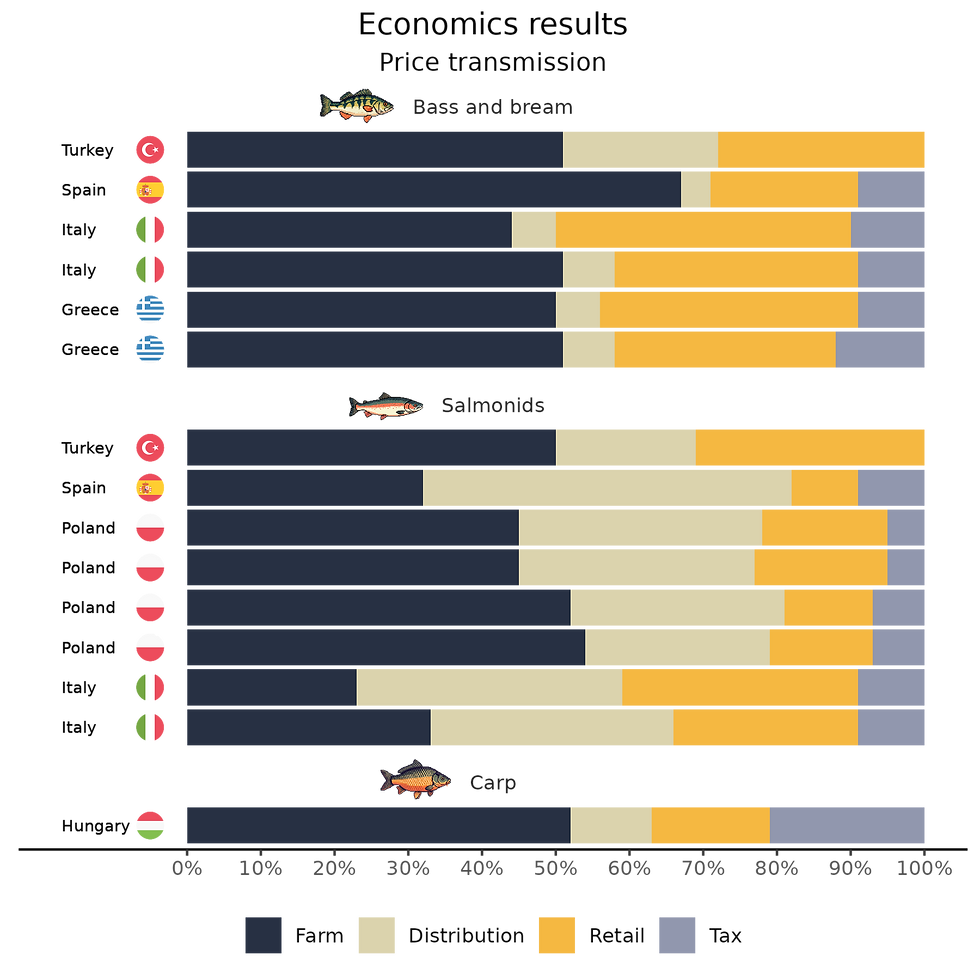
The following table summarises qualitative findings related to price transmission and market power.
In general, the take-home messages are as follows:
For Atlantic salmon, farmers tend to have power to set prices. There is generally a high degree of price transmission from farmers to retailers. However, this power is not total. Retailers in large consumer markets, such as the UK, France, and Germany, have a greater ability to set prices. There are also exceptions for some product types (e.g. fillets and highly processed products) due to additional processing steps.
For bass and bream, retailers generally set prices, and farmers are forced to accept these prices.
Table 1. Findings from studies on price transmission and market power
1.4. Market integration and consumer dynamics
Market integration and consumer purchase behaviour gives us information about how the market will respond to changes in price or supply of fish. For example, if we suspect that a particular policy intervention could increase the price of fish, we can only develop a comprehensive understanding of the net effect across all fish if we understand whether and how consumers are likely to change their purchases.
In general, the take-home messages are as follows. For salmonids:
Farmed salmon and wild salmon are substitutes.
Fresh salmon and frozen salmon are substitutes.
Salmon and some (but not all) other fish species are substitutes.
Portion trout with white meat as a substitute for wild-caught fish of other species, but not for salmon.
Most studies found that the demand for and production of salmon is relatively unresponsive to price in the short run but comparatively more responsive to price in the long run. An exception is one study from Germany, which found that demand for salmon is indeed responsive to price.
For bass and bream:
The production method (farm vs wild-catch) usually matters. In Spain, Italy, and France farmed bass and bream and wild-caught bass and bream are not substitutes. In contrast, studies on Turkish markets have found conflicting results. One study (out of a total of four) in Spain also found that farmed and wild-caught bass and bream are indeed substitutes.
The country of origin usually matters. In Spain and France, imported bass and bream and domestic bass and bream are not substitutes. In Spain, Greek imports and Turkish imports are not substitutes. However, in Italy, Greek imports and Turkish imports are indeed substitutes.
The table below summarises the key findings by study.
Table 2. Findings from studies on market integration
1.5. (Not much) evidence of economic costs of fish welfare improvements
A key take-home message is that no studies have calculated the real-world economic effects of an animal welfare improvement after implementation.
There are several studies that have used simulations and modelling to project the economic effects of welfare improvements. These studies are generally based on real-world costs of equipment but remain hypothetical simulations.
Studies have examined three welfare improvements:
Electrical or mechanical stunning to reduce suffering at slaughter (Eurogroup for Animals, 2024; European Commission, 2017; Ren Ryba, 2023b, 2023a). The most recent of the studies examined 17 different industries and found that the average costs of stunning, per kg of production,are 0.33 €/kg for carp (13%), 0.06 €/kg for seabass/seabream (1%), and 0.10 €/kg for trout (3%) (Eurogroup for Animals, 2024).
Carbon dioxide stripping to improve water quality in Atlantic salmon hatcheries (Noble et al., 2012). Using hatcheries in Norway, the authors calculate that the result of introducing carbon dioxide stripping would improve productivity and thus be a net profit increase for hatcheries.
Demand feeders to reduce fin damage during smolt production of Atlantic salmon (Stewart et al., 2012). Using smolt farms in the UK, the authors calculated that introducing demand feeders would increase the costs of production by around 0.04 EUR/kg (in 2025 terms).
Previously, studies have also examined the economic effects of using triploid rather than diploid salmonids, though this is less relevant to us—at least in the UK, almost all farmed Atlantic salmon are triploid (Munro, 2021).
2. Special sections
2.1. Juveniles, hatcheries and nurseries
This section contains information specific to juvenile production in hatcheries and/or nurseries.
Economic information about hatcheries
Norway: Recent government data shows that the industry-wide profitability (as a percent of revenue) of Norwegian salmonid hatcheries is around 11% (Fiskeridirektoratet, 2024). Costs of production were 18% feed, 13% stocking, 14% labour, and 54% other costs (Fiskeridirektoratet, 2024).
UK: A study of two salmonid hatcheries in 2012 found that the profitability (as a percent of revenue) was around 13% (Stewart et al., 2012). Costs of production were 11% feed, 17% stocking, 20% labour, and 51% other costs (Stewart et al., 2012).
Turkey: A study of European seabass and gilt-head seabream hatcheries in 2002 found that the profitability (as a percent of revenue) is about 17% (Buke, 2002).
Fingerling and juvenile numbers
Norway in 2023: 307 million Atlantic salmon released and 17 million rainbow trout released (Sommerset et al., 2024).
UK in 2021: 51.2 million Atlantic salmon smolts put to sea (Munro, 2021).
Spain in 2015: 41.7 million European seabass, 32.4 million gilt-head seabream, and 10.3 million rainbow trout (Muñoz-Lechuga et al., 2018)
Turkey in 2001: 50 million European seabass (Buke, 2002). In 2021, the theoretical capacity of Turkish marine hatcheries was estimated at 776 million European seabass and gilt-head seabream fry (WWF, 2021).
Italy: An average of 2,714 juveniles at a body weight of 5 grams are required to produce 1 ton(ne) of fish (Zoli et al., 2024).
Malta: Peak production was 2.5 million gilt-head seabream fry per year, most of which were exported to other European countries (Holmer et al., 2008).
Other findings
In Turkey, 5 g salmonid fingerlings cost around 0.01 USD per fingerling as of 2006 (Cinemre et al., 2006).
Rainbow trout in Spain: The viability of eggs was estimated at 95% (Sanchez-Matos et al., 2023).
In Turkey, one study identified 22 hatcheries for marine fish in 2017 (Hekimoglu et al., 2018), which can be compared with 15 hatcheries for European seabass in 2001 (Buke, 2002).
Turkey exports European seabass and gilt-head seabream fry to Gulf countries (e.g. Saudi Arabia and Oman) and Tunisia (WWF, 2021).
In Bulgaria, juvenile production is very low (9 farms in 2019), and there is a significant need for imported eggs (Uzunova et al., 2023)].
Live Artemia crustaceans are used as food in European seabass hatcheries (Buke, 2002).
2.2. Cleaner fish
This section contains information specific to cleaner fish.
The two key species groups are lumpfish or lumpsucker (Cyclopterus lumpus) and wrasse, including Ballan wrasse (Labrus bergylta) and other wrasse species. Lumpfish are generally farmed, while wrasse are either farmed or caught from the wild (Bolton-Warberg, 2018).
Numbers
Norway: Around 50 million cleaner fish are deployed on farms in Norway each year (Barrett et al., 2020). A report for 2023 estimated 34 million cleaner fish deployed in 2023 (Sommerset et al., 2024). The species are lumpfish (56%), goldsinny wrasse (18%), corkwing wrasse (17%), Ballan wrasse (5%), and other wrasse (4%) (Barrett et al., 2020). Of the 37 million cleaner fish used in 2016, 16 million originated from cleaner fish farms in Norway and others originated from Norwegian and Swedish wild-catch fisheries (Treasurer, 2018).
UK: In 2017, 4.3 million lumpfish and 500,000 Ballan wrasse were deployed on farms (Treasurer, 2018). This includes roughly 2.5 million lumpfish from eight hatcheries, 250,000 Ballan wrasse from three hatcheries, and 1–2 million wild-caught wrasse (Treasurer, 2018).
Republic of Ireland: In 2015 and 2016, 350,000 cleaner fish were produced from 1.4 million eggs (including eggs sourced from Ireland, Scotland, and Norway) (Treasurer, 2018). From 2015 until around 2018, over 300,000 hatchery-reared lumpfish and over 600,000 wrasse were deployed on Irish salmon farms (Bolton-Warberg, 2018).
Biology
Survival rates: In UK hatcheries, survival rates are 1–20% for Ballan wrasse and over 50% for lumpfish (Treasurer, 2018). In Irish hatcheries, lumpfish survival rates are around 60% (Treasurer, 2018).
Duration: In the UK, Ballan wrasse are weaned after 30–60 days and lumpfish after 4–12 days (Treasurer, 2018). In Norway, lumpfish seem to remain on salmon farms for between 60 and ~200 days (Reynolds et al., 2022). There is large variation; one source reports an average of 68 weeks, though this is based on the duration of lice reporting rather than the survival of cleaner fish per se (Barrett et al., 2020). It appears that cleaner fish almost always die on salmon farms, rather than at harvest (Poppe, 2017).
Body size: In Norway, lumpfish seem to be transferred from suppliers to commercial farms at around 35 to 150 g (Reynolds et al., 2022). Treasurer et al provide biological growth curves for lumpfish farmed in Ireland (Treasurer, 2018).
2.3. Sturgeon and caviar production
This section contains information specific to sturgeon and caviar production.
Key findings
For global sturgeon meat production in 2016, the most common species were Acipenser baerii (40% of reported production), two hybrids (Huso dauricus × Acipenser schrenckii and A. baerii × A. schrenckii; 36% together), and A. schrenckii (10%) (Bronzi et al., 2019).
For global caviar production in 2016, the most common species were A. baerii (31%), A. gueldenstaedtii (20%), the hybrid H. dauricus × A. schrenckii (13%), A. transmontanus (12%), and A. ruthenus (5%).
Sturgeons are farmed using several different production methods: flow-through and/or RAS (68% of global reported production), cages (18%), ponds (7%).
Sturgeon females take 8–10 years to reach sexual maturity. As such, sturgeon farms typically begin producing meat (from males) before caviar is produced. A farm produces meat and caviar typically in a 20:1 ratio (i.e. 20 tonnes of meat for 1 tonne of caviar) (EUMOFA, 2023a).
Sturgeon and caviar extracts are also used in beauty products and high-end luxury products (EUMOFA, 2023a). There is also a market for sturgeon leather, as well as other products like sturgeon fins, swim bladders, and (bizarrely) sturgeon genitalia.
Sturgeon meat is most popular in Russia and China, and European producers frequently export to these countries. Sturgeon is also consumed in eastern European countries like Bulgaria, Ukraine, Serbia, and Romania (EUMOFA, 2023a).
Caviar is usually sold for high-end consumption in Michelin-starred restaurants, specialty retail shops, high-end hotels, first- and business-class services in airlines, and cruise ships (EUMOFA, 2018). Caviar produced in the EU is frequently exported to the US, Japan, the United Arab Emirates, Hong Kong, and Switzerland. Increasing consumption from China has been driving a global increase in caviar production as a whole.
The following graph shows estimated production by country, using data from 2020 and 2016 (we report here only the countries in-scope for our review) (Bronzi et al., 2019; EUMOFA, 2023a). In total, these countries produce around 3,200 tonnes of sturgeon meat and 152 tonnes of caviar in one year.
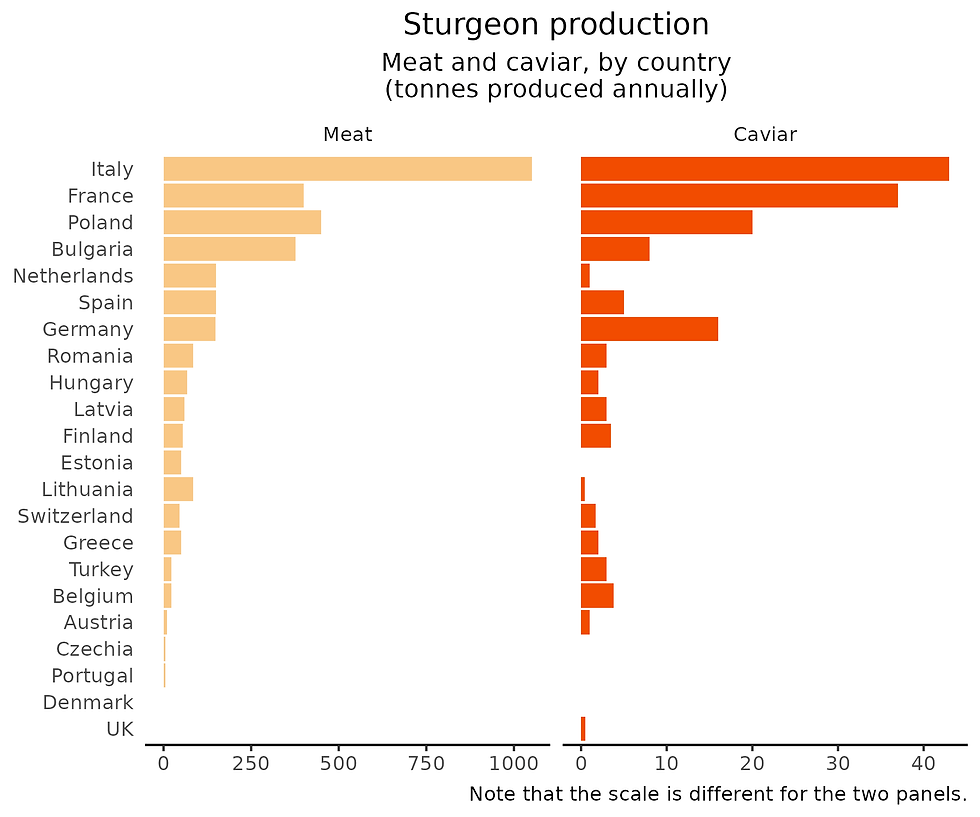
3. Other useful insights
This section contains a list of qualitative findings that we think might provide additional insight into aquaculture production and markets.
3.1. General insights
Inflation and global instability (e.g. due to the COVID-19 pandemic and Russia's invasion of Ukraine) has appeared to cause a net decrease in fish consumption by volume in Europe (EUMOFA, 2023b). The total volume of fish consumed dropped by 17% in some countries between 2021 and 2022. This appears to be due to the increased cost of living and the increasing prices of fish specifically; in response to these trends, consumers have appeared to reduce their fish purchases and to opt for smaller portion sizes (EUMOFA 2023b; Directorate-General for Maritime Affairs and Fisheries 2021).
In the EU, farmed fish consumption consists of roughly one-third domestic (EU) production and two-thirds imported fish (EUMOFA, 2023b).
Farmers seek certification from certifiers such as Aquaculture Stewardship Council for a number of reasons (Olsen et al., 2021). Some farmers emphasise the higher market price. However, it is more common for farmers to point towards greater market access and reputation gains.
In some cases, the country that farms fish is the same country that processes and prepares the fish for sale (Graziano et al., 2018). In other cases, fish are exported for value-addition to countries with lower labour costs (especially Poland).
In some countries, fish farmers—particularly small-scale fish farmers—work part time and also derive income from other sources as in Germany (Lasner et al., 2020), Croatia (Pećarević et al., 2023), and Finland (Salmi et al., 2003).
French aquaculture production cannot easily expand in terms of space due to land ownership and legal restrictions (Regnier & Bayramoglu, 2017).
Organic production is quite low, consisting of 6.4% of EU aquaculture production; however, less than half of this percentage is finfish (EUMOFA, 2022a) The main EU producers of organic finfish are Ireland (salmon), Italy, France (trout), and Spain (sturgeon) (EUMOFA, 2022a). The market share of organic finfish appears to be nil in France (Chen XianWen et al., 2015) and around 0.3% in Germany (Ankamah-Yeboah et al., 2017). In Greece, only around three European seabass and gilt-head seabream farms are certified as organic (Perdikaris & Paschos, 2010). In Lithuania, around 15 farms are engaged in organic production (Pileičikas, 2010). In Italy, around 1% of aquaculture production (including shellfish) is organic (Sicuro, 2019).
Likewise, production in integrated multi-trophic aquaculture (IMTA) systems seems extremely low. In Europe, we are only aware of two commercial-scale IMTA farms (one in Northern Ireland and one in Portugal) (Costa Rainha, 2018).
A hypothetical online experiment of 1,236 German consumers found that consumers are willing to pay more for organic farmed trout compared to non-organic farmed trout (Ankamah-Yeboah et al., 2019). Information about the animal welfare benefits increased the willingness-to-pay.
The effects of climate change vary by country and species (Cubillo Alhambra Martínez et al., 2021). Simulation models suggest that farmers in some contexts (e.g. European seabass and gilt-head seabream farmers in Turkey and the Canary Islands) may see increased profits due to the effects of climate change (CERES 2020; CERES 2020a; Taylor et al., 2019).Other farmers may see decreased profits (Taylor et al., 2019).
3.2. Bass and bream
The physical, economic, and geographical details of European seabass and gilt-head seabream farms vary by country (Pelt et al., 2024): "Greece and Türkiye tend to have fish farms very close to the shoreline. Half of Greece’s fish farms are located within 170 metres of the shoreline, while half of Türkiye’s fish farms are located within 180 metres of the coastline. In contrast, Italy and Spain tend to have fish farms much further from the shoreline. Half of all fish farms in Italy are located within 990 metres from the coastline, while half of Spain’s fish farms are located within 1,600 metres of the shoreline."
Bass and bream are typically fed commercial feed, but they may also eat bacteria, plankton, and small plants and animals that they can access (Briones-Hidrovo et al., 2023).
3.3. Salmonids
The Norwegian salmon industry has large market power over buyers; there is more demand for salmon than there is production, so many buyers (e.g. Polish distributors) are forced to take whatever they are offered by the salmon industry (Voldnes et al., 2023).
Over time, Norwegian salmon production has become more productive (more salmon produced for a given set of inputs), but this productivity has recently slowed down (Asche et al., 2013). A similar trend is observed for the production of salmon juveniles in hatcheries (Sandvold & Tveteras, 2014). This indicates two things: firstly, the increasing production of salmon may be attributed to higher demand rather than higher supply; and secondly, production may soon become limited by the availability of production sites (Asche et al., 2013). In the EU, demand for salmon has changed significantly over time. However, the cause behind a majority of this change in demand is unknown (Brækkan et al., 2018).
The mean weight of salmon harvested in Norway has decreased by around 310 g (6.6%) between 2012 and 2021 (Barrett et al., 2022). This is partially attributed to the physiological effects of delousing treatments (Barrett et al., 2022).
Volatility in the price of salmon seems to be primarily caused by volatility in the availability of key inputs, especially feed (Oglend, 2013). Recently, the relationship between feed price and salmon price has become very close, indicating that changes in feed prices are reflected in the salmon price (Asche & Oglend, 2016). However, there are mechanisms to level out this price volatility. Norwegian salmon exports are often traded using fixed price contracts (e.g. 25% of Norwegian salmon exported to France in 2006) (Larsen & Asche, 2011). Contracts are negotiated as infrequently as once per year. This creates a wedge between salmon export prices and market prices, which reduces price transmission.
Salmonid consumption might be slightly higher around Christmas and Easter (Asheim et al., 2011).
The Norwegian government appears comfortable taxing fish farmers; in 2022, the Norwegian Ministry of Finance introduced a rent tax (Misund et al., 2024).
In the UK, less than 25% of feed originates from domestic crop production (Newton & Little, 2018). In particular, almost 50% is imported from South America.
In the UK, almost all farmed salmon are triploid (Munro, 2021).
In some countries (e.g. Denmark), trout is occasionally raised to large sizes (e.g. 5 kg) for the production of roe or trout caviar (Animal Ask, 2023; Skov et al., 2020). EU member states produced around 1,000 tonnes of trout roe in 2020 (EUMOFA, 2022b).
The seafood production sector struggles to recruit and retain workers, as found for the entire seafood supply chain in the UK (Witteveen, 2023) and juvenile fish production in Greece (Pyrgilis et al., 2022).
In 2010, the Chinese government applied import restrictions against Norwegian salmon in response to the Nobel Peace Prize being awarded by the Norwegian Nobel Committee to a Chinese human rights activist (Graziano et al., 2018).
3.4. Carp
Carp production in Bulgaria may be underreported (Hadjinikolova et al., 2010).
In some countries, carp is overwhelmingly consumed at Christmas (e.g. 90% of carp sales in Czechia and Poland), though this effect is less drastic in other countries (e.g. 30% in Hungary) (Edwards, 2007; Jakub Stencel & Weronika Zurek, 2022).
Small-scale carp farmers in Poland often farm crops to use as feed for the carp (Lasner et al., 2020).
Carp farmers face predation by wild animals. Wild otters predate on carp in some countries (e.g. Austria, Czechia) (Bohm et al., 2013; Myšiak et al., 2013). Many strategies are used to avert this, including electric fences and specific pond management practices. Compensation was previously provided by governments, but this has become less common. Wild cormorants also predate on carp (e.g. Lithuania) (Pileičikas, 2010).
4. Biology of production
We also encountered many sources that gave us country- and species-specific information on key biological statistics, including harvest weight, mortality, and the duration of the production cycle. Combining these statistics is naturally a looser and less formal process than combining economic statistics. Like with economic parameters, there is lots of variation in biological statistics across farms and within farms.
Here, we give some general indications on key biological statistics. These should be considered as approximate, indicative values, not precise averages. To interrogate parameters in more detail, see the "results - biol" tab in the main spreadsheet (linked below).
Also note that the distinction between the hatchery/nursery phase and the grow-out phase can be blurred, especially for Atlantic salmon.
In the table below, mortality is given as a cumulative proportion; duration is given in months; and body weight is given in grams.
Table 3. Biological statistics of production
Note: mortality is given as a cumulative proportion; duration is given in months; and body weight is given in grams.
S. Supplemental information and methodology
S.1. Link to full results and code
We intend for the document that you are reading to be a stand-alone resource with which fish advocates can understand this systematic review's methodology, results, and implications for advocacy. However, you may be interested in detailed results (e.g. for further research or to interrogate particular data points). In this case, please feel free to view these two resources:
The Google spreadsheet into which we extracted data from papers during the full-text review is available here. The "readme" tab will help you get oriented.
The Github repository, which contains R code and supporting data files, is available here. We intend this repository to be accessed by users who are comfortable reading R code; however, we have endeavoured to explain the purpose of each R file in the methodology below.
In the following methodology section, we frequently refer to both the Google spreadsheet and the Github repository.
When we refer to a spreadsheet tab, this always means a tab within the Google spreadsheet. Such references are highlighted in the below text in green.
When we refer to a specific file, this always means a file in the Github repository. Such references are highlighted in the below text in gold.
Note that we have not shared the Zotero library with the full-text PDF files, as sharing this library freely online would probably violate some form of copyright law.
S.2. Coverage
The following graphic shows the coverage of economic data that we obtained in our systematic review, by country and species group.
This only refers to formal economic parameters, as visualised in the main graphs in the results section of this report. Some of the cells that are defined as "no data" or "no industry" did in fact yield biological or qualitative information.
The classification of "no industry" is defined, purely for the purposes of this particular graph, as industries with fewer than 1 million fish slaughtered per year. These industries were still included in the scope of the systematic review as a whole.

S.3. Methodology
S.3.1. Scope of this review
In our systematic review, we used the following inclusion and exclusion criteria.
Table S1. Inclusion and exclusion criteria for systematic review
* Initially, we considered including the United States and Canada in our full analysis, and these two countries were included in the data collection. However, after data collection, we decided to limit our formal analysis to Europe. We have kept the papers that we found on the United States and Canada for a future project.
** We decided to limit our literature search to records published from the year 2000 onwards. This was an ad hoc decision, made at the relatively late stage of retrieving full texts. We found that most records from before 2000 were not available online, and we believe that much of the published research from before 2000 will be inapplicable due to significant geopolitical and economic changes in the 1990s and earlier.
*** Juvenile fish, cleaner fish, and sturgeons are addressed in specific sections of this report, rather than being incorporated into the main calculations and visualisations. This is due to limited data and the unique nature of these industries.
S.3.2. Systematic review flowchart
The following flowchart provides a visual summary of the inclusion and exclusion of records during our systematic review.
Note that the 571 records that proceeded to data extraction include some records that were out-of-scope, duplicates, or flagged for fish welfare data only, but where this was only noticed during data extraction.
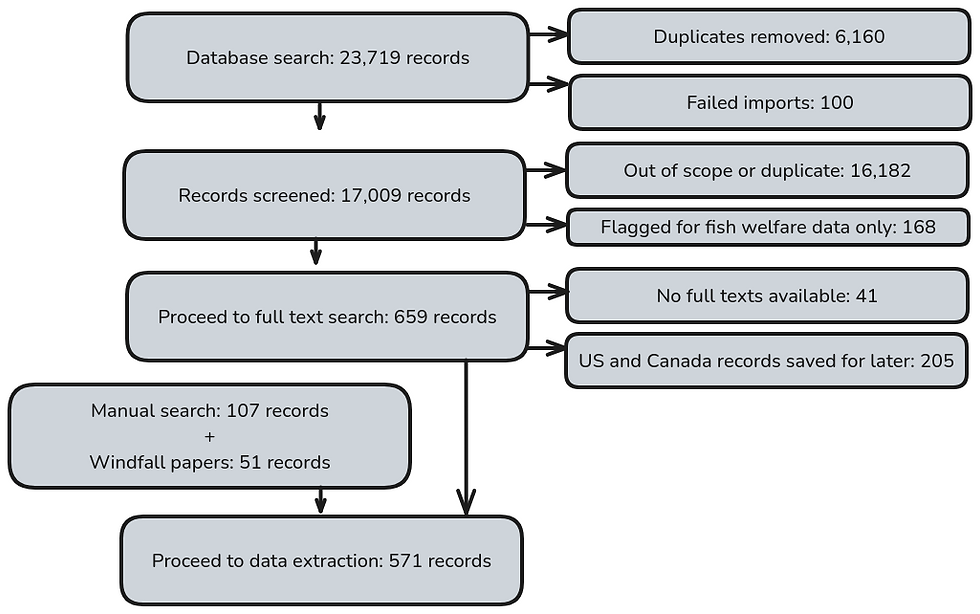
S.3.3. Statement on generative AI
We are cautious about the limitations of generative AI and hesitant to use it in research. As such, very few of the automation tools used in this systematic review involved generative AI or large language models (LLMs).
However, there were three small steps in our methodology where we did use the generative AI model Gemini. For these three steps, we were conscious to reduce the chance of hallucination and other errors harming the integrity of the dataset. We only used LLMs to process data that is trivial (e.g. keywords that we came up with) or publicly accessible (e.g. titles and abstracts of papers).
The first was the sorting of keywords, which were themselves generated without the use of LLMs, into the order necessary for the construction of a boolean search string. Since there were over 100 keywords at this stage (naive + extend keywords as defined below), sorting these keywords with LLM-assistance was a useful time-saver. The results of this step were manually checked.
The second was the translation of the handful of abstracts and titles of papers that were in non-English languages and where Google Translate failed to translate (usually due to an unrecognised language code). This latter step involved 25 abstracts and titles (from the 331 papers that were flagged as non-English of a total number of 17,010 papers that proceeded to the screening stage). For transparency, all translations that used a LLM were flagged as such in the resulting dataset, and the original non-English titles and abstracts were retained as a backup option.
The third was extracting the country and species group on which each record focused. This step involved the 725 records that proceeded to full-text screening (see file full_text_review/fulltexts_combined_databases_and_manual_searches_analysedbygemini.csv and specifically the data columns llm_country and llm_species). This step was performed immediately before full-text screening, and every result was checked manually when the full texts were reviewed.
We also used Imagen 3 to generate three small pixel illustrations of fish, which we modified and incorporated in the visualisations in this report.
S.3.4. Search terms
We constructed search terms for our systematic review using keywords derived from three sources. In total, our search term consisted of 1,996 keywords, though some database searches were conducted with a subset of these keywords. The replicable code for generating this set of keywords is saved as 01_keywords.R in the Github repository.
The three sources for keywords were:
Naive: We manually specified search terms that we brainstormed ourselves, relating to geography (e.g. country names), industry (e.g. terms relating to fish aquaculture and fish welfare), economic parameters (e.g. economic, cost, price), binomial species names, and common species names. There were 144 terms in this category.
Extend: We automatically extended a subset of the naive terms (the terms relating to industry and economic parameters, but not geographies or species names) using the R package litsearchr (Grames et al., 2019). This process involved conducting a naive database search of Web of Science, extracting the first 1,000 results, and automatically processing the results to derive an additional set of relevant, extended search terms (Grames et al., 2019). This resulted in an additional 94 terms in this category. These 94 terms were sorted into the predefined categories (i.e. whether they related to industry or economics) using Gemini.
Translate: We automatically translated a subset of the naive terms (the terms relating to geography, industry, common species names, and economic parameters, but not binomial species names) using DeepL via the R package deeplr (Zumbach & Bauer, 2023). We used DeepL here, despite its limited free usage, because accuracy is important for specifying search terms. These terms were translated from English into each of Bulgarian, Czech, Danish, Dutch, English, Estonian, Finnish, French, German, Greek, Hungarian, Italian, Latvian, Polish, Portuguese, Romanian, Slovak, Slovenian, Spanish, Swedish, Lithuanian, Turkish, and Norwegian. This resulted in an additional 1,758 search terms in this category.
For the final search term strings, which are very long and unwieldy, see the spreadsheet (tab "searches").
S.3.5. Databases and other data sources
The databases that we searched are listed in the table below. We aimed to conduct searches in a range of databases covering multidisciplinary research, economics, agriculture, grey literature, and non-English literature. For the full details of searches, including dates of searches, search terms and numbers of results, see the spreadsheet (tab "searches").
Table S2. Database searches
* maximum search terms are mostly taken from searchsmart.org
** the components of search terms ("native", "extend", and "translate") refers to the dot-point list in the above section
Please note that our web builder does not allow us to upload this report in its entirety. To continue reading from this point, you will need to download the pdf version of the report using the button below.
Commentaires